What is Geoexchange?
To introduce this topic, let’s start off with an interesting fact: Anything with a temperature above absolute zero (-273°C) has heat energy in it. As well, anything warmer than absolute zero has stored energy. Geoexchange technology captures and uses stored heat from the ground for providing energy-efficient heating and cooling for buildings ranging from single family residential homes to the largest institutional and commercial buildings. What makes geothermal energy even more interesting, is that this isn’t new technology. The technology has existed for decades and has been improved, and adapted upon. With wise and thoughtful design adapted to specific site conditions, geoexchange systems can be cost-effective, and can be adapted for homes, commercial and institutional buildings, and industrial process applications.
Why is there a growing interest in Geoexchange?
Geoexchange heating and cooling (also sometimes referred to as ground source heat pump technology, or geothermal heat pump technology) is a technology option often considered as a part of electrification strategies for decarbonizing heating loads. In many applications, well-designed geoexchange systems can eliminate, or nearly eliminate, carbon emissions associated with building heating and cooling.
How does it work?
The geoexchange principle relies on a specific application of the refrigeration cycle for transferring heat from one place to another. By moving heat instead of converting chemical energy into heat (e.g. fossil fuel combustion), geoexchange systems can often provide space and/or process heating (and cooling) in a much more energy-efficient manner than conventional heating or cooling systems. Geoexchange heat pumps can extract energy from low-grade heat sources (at temperatures below 5°C) and “concentrate” the heat to a higher temperature for delivery to a heated environment. Hence, heat pumps can be coupled to a ground heat exchanger (often consisting of buried piping within the earth) so that the ground becomes the heat source for the system.
The energy-efficiency of a heat pump is dependent on the temperature of the source/sink that it is coupled with. In heating mode, the energy-efficiency increases as the source temperature increases. In cooling mode, the energy-efficiency increases as the rejection temperature decreases. Because the undisturbed earth temperature is warmer than the average winter air temperatures and cooler than average summer air temperatures, the ground makes for an attractive heat source for winter heating and heat sink for summer cooling.
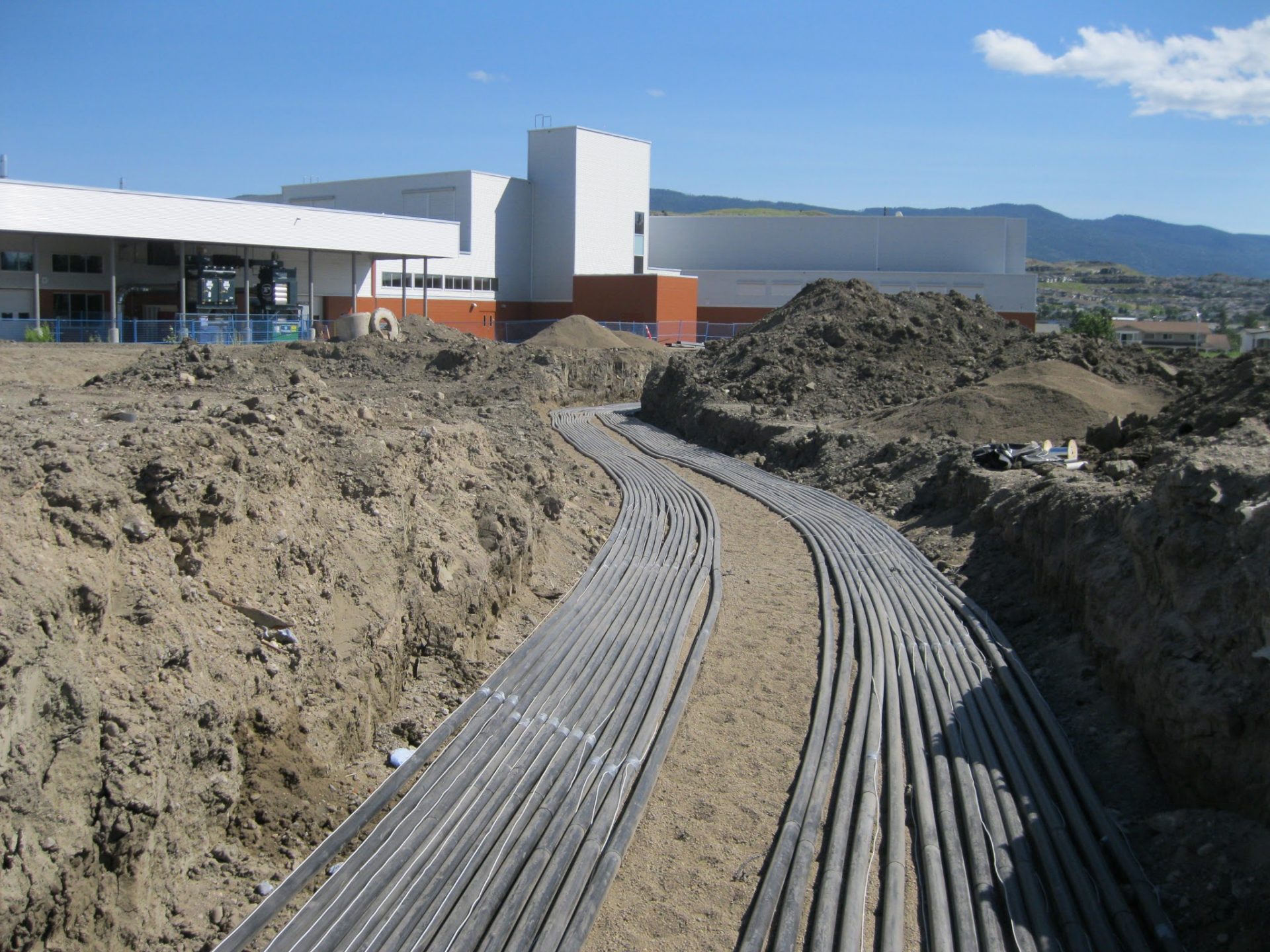
Geoexchange Harnesses Renewable Energy
The ground energy harnessed by geoexchange systems is renewable energy. Most of the energy captured by geoexchange is solar energy absorbed by the earth’s crust while a smaller portion is core geothermal energy. In the geologically diverse western cordillera of BC, the core geothermal component varies from site to site and in some cases anomalously high geothermal gradients may cause the core geothermal component to be significant. Although electrical energy is required to drive the heat pumps, carefully designed geoexchange systems are capable of achieving coefficients of performance (COP) exceeding 3.5 in heating mode (COP is the ratio of the total heat delivered by the heat pumps relative to the electrical energy required to drive the heat pumps). At a COP of 3.5, 71% of the total heat delivered is renewable energy transferred from the ground and the remaining 29% is derived from grid electricity.
Ground Heat Exchangers (GHX)
Ground heat exchangers (GHXs) can take many forms, but all forms belong to one of two broad categories:
- Closed-loop GHX systems which rely on conductive heat transfer between the earth and a network of piping through which a heat transfer fluid is circulated in a closed-cycle. Examples of closed-loop GHX include vertical closed-loop borehole systems and horizontal trenched piping systems.
- Open-loop GHX systems which rely on the actual transfer of groundwater or surface water through the geoexchange cycle.
The four most common types of GHX are:
- Vertical borehole GHX – Closed-loop (V-GHX). This method involves drilling a network of boreholes that are each typically 50 to 250 m deep (depth often depending on site-specific constructability factors). Two pipes are placed in each borehole with a u-bend connection at the bottom and heat transfer fluid is circulated through the borehole network. This is the most versatile method and can be adapted to the widest range of settings. However, the vertical borehole GHX method is typically the most expensive option when other options are available.
- Horizontal trenched GHX – Closed-loop (HT-GHX). This method involves installing heat exchange pipes in trenches that are typically 1.5 to 3.0 m deep (depending on climate and soil conditions). Without the depth dimension, the horizontal closed-loop method requires a much larger footprint area to generate the same heat exchange capacity as a drilled vertical borehole system. Consequently, horizontal systems are limited to applications where large open areas are available (such as for schools with large play fields or agricultural operations with large areas of surrounding cultivated land).
- Horizontal directional-drilled GHX – Closed loop (HDD GHX). The horizontal directional-drilled (HDD) method involves installing heat exchange pipes in drilled horizontal boreholes that are typically 5 to 10 m below the ground surface. Because the boreholes are drilled below the surface, this method results in much less ground disturbance than the trenched method. As with the trenched horizontal systems, the horizontal closed-loop method requires a much larger footprint area to generate the same heat exchange capacity as a drilled vertical borehole system. The HDD method can be well- suited where upper soils consist of laterally continuous fine-grained (easily-drilled) soils (particularly when deeper soils are unsuitable for cost-effective vertical drilling), or where extensive surface disturbance would disfavour trenched methods.
- Groundwater Production/Injection Well Pair – Open loop (GW-GHX). Groundwater open-loop systems typically move groundwater from a producing well (or wells) through a heat exchanger and then return the groundwater (at a lower temperature in heating mode or a higher temperature in cooling mode) to the aquifer by injection well(s). In certain settings, these systems can be very cost-effective where high rates of high-quality groundwater can be produced sustainably. However, appropriate site conditions for medium scale open-loop groundwater systems are relatively rare, and conditions suitable for large-scale open-loop groundwater systems are rarer still. Furthermore, these systems typically require more diligent attention to maintenance, incur higher maintenance costs than closed- loop systems (particularly if the groundwater is highly mineralized or is otherwise not of high quality), require careful highly site-specific design, and require a licensing process that involves consideration of affects to existing groundwater users in the area. Large GW-GHX systems may also require navigating an environmental assessment process often including groundwater flow and thermal simulation modelling if the rate of groundwater production is large (in British Columbia an environmental assessment is typically triggered for projects involving groundwater production exceeding 75 L/s).
Systems comprised of a network of vertical boreholes are the most common type of geoexchange system – but sometimes other types of systems are suited depending on site-specific setting. (Source: Natural Resources Canada)
Common Misconceptions
There is a common misconception that the temperature of the soil/rock surrounding a closed-loop GHX remains constant despite the transfer of heat in and out of the ground through the GHX. As a result, there is a widely-accepted perception that geoexchange systems always operate at a consistent performance level because they supposedly tap an “unlimited availability of heat at a constant ground temperature”. Unfortunately, this perception is false and misleading and often leads to inappropriate applications of geoexchange. In fact, the ongoing thermal interaction between the heat pump system and the closed-loop GHX causes the temperature of the soils that are thermally coupled with the GHX to fluctuate. The GHX temperature varies in response to the “rate” (power) and the cumulative “quantity” (energy) of heat extracted from (or rejected into) the earth and can be quite sensitive to the relative balance of the annual heating and cooling loads. Temperature fluctuations are damped as a function of site-specific soil thermal properties and the size and configuration of the GHX. As a general rule, the better the soil thermal properties, the bigger the GHX, and the more balanced the annual heating and cooling loads, then the more stable the GHX operating temperatures.
Proper accounting for these relationships is important for selecting appropriate GHX option(s) and it is crucial for supporting effective and sustainable design of the selected option.
Relative Balance of Heating and Cooling Loads
Closed-loop type of geoexchange systems operate most effectively when they serve both heating and cooling loads. In cooling mode, the heat is transferred into the ground causing the soils in proximity to the GHX to warm and store heat. Then in the winter cooling mode the stored heat can often be re-captured from the ground for heating purposes. In this manner the heat is essentially “recycled” from season to season, resulting in considerably less strain on the GHX, and allows the GHX to behave more as a store and less as a source of heat.
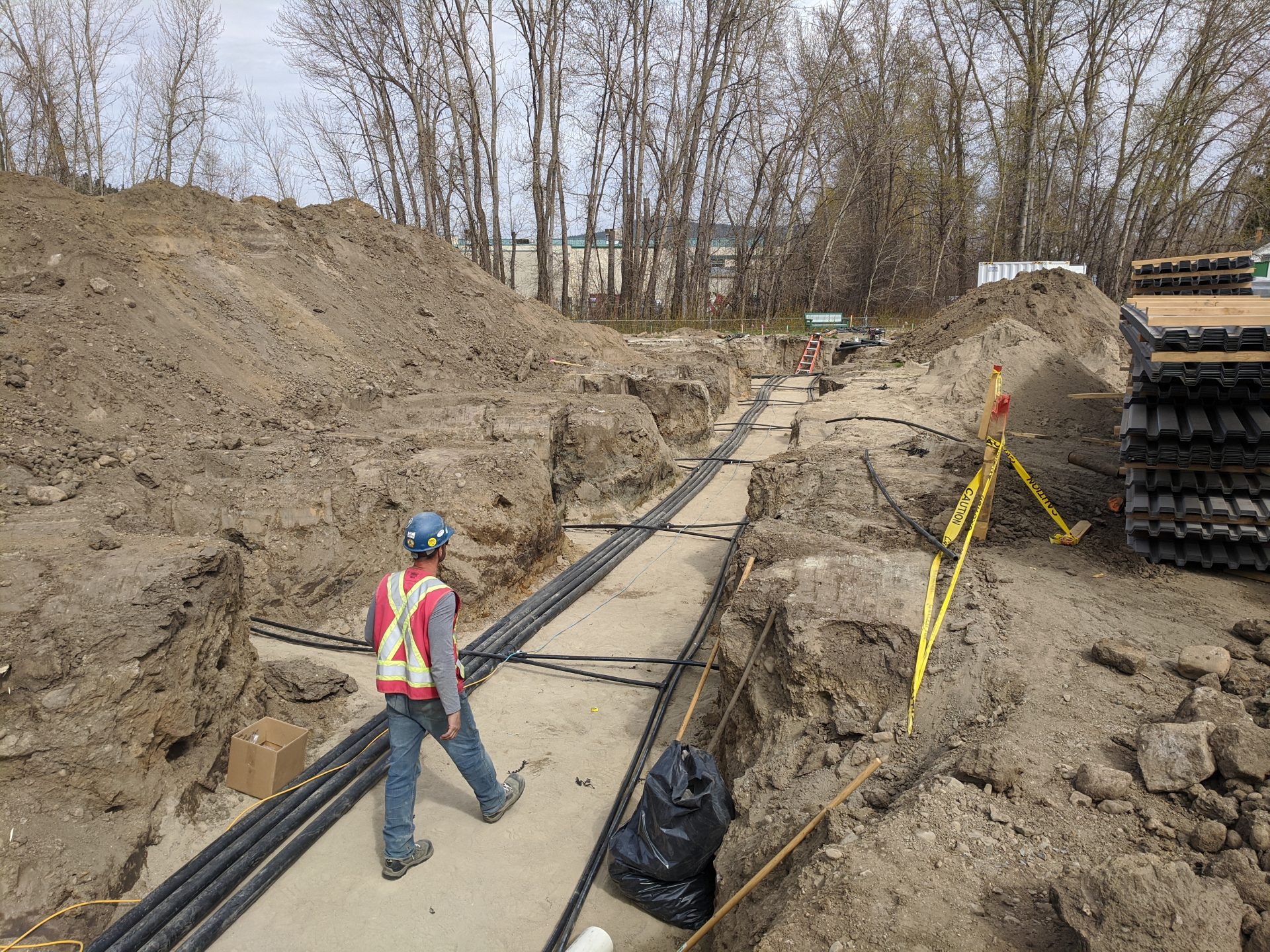
With careful design, geoexchange systems can be designed to sustainably serve one-way heavily dominant heating loads. For closed-loop type of systems, bigger GHX systems are typically required to serve one-way loads (e.g., more boreholes or deeper boreholes), and the spacing between the boreholes needs to be significantly increased so that the GHX is in thermal connection with a much greater mass of soil from which to transfer heat. For open-loop type systems serving one-way loads, greater attention to the separation between extraction and return points is required.
Importance of the Options Analysis Step
The cost for constructing the GHX portion of geoexchange systems varies by a factor of nearly ten-fold per unit capacity.
The large range is attributed to many factors including:
- GHX type (different installation methods with widely varying cost).
- Relative balance of heating loads (unbalanced loads require bigger GHX that are more expensive).
- Site-specific soil and rock conditions (localized ground conditions can be challenging for drilling or trenching which can significantly escalate cost, and because geological and hydrogeological conditions vary more widely in BC than in other regions, this factor has a more prominent effect in BC than elsewhere).
- Procurement processes (amount and type of information provided to bidders and the type of process used can significantly affect cost).
An objective evaluation of site-specific conditions and available site-specific options at an early stage in the planning process can help identify unique geoexchange opportunities (improving technical performance, reducing cost, or managing risk), and can help identify constraints or limitations that may impede suitability for geoexchange adoption.
What are geoexchange solutions best suited for?
Along with air-source heat pump systems, geoexchange heat pump systems will play a crucial and growing role in efforts to decarbonize building heating and cooling. Geoexchange is particularly suited in the following scenarios:
- Colder climate locations where air-source heat pumps are unsuitable or less effective. Geoexchange can decarbonize heating in northern BC, such as Prince George and Dawson Creek.
- Settings where the ground can be used to store rejected summer heat, or sources of waste heat, for uptake for winter heating.
- Settings where unique cost effective opportunities for ground heat exchange are available.
- Settings where silent outdoor operation is desired (in contrast with air-source heat pump systems that often generate considerable noise).
The Importance of a Thorough Design
While geoexchange heat pump systems aren’t particularly complex, they do require thoughtful and thorough design, and particular care in optimizing the integration of the geoexchange subsystems including:
1) Ground Heat Exchanger
2) Heat Pump Plant
3) Distribution System.
Heat pumps deliver heat differently than combustion systems and the design of the systems needs to take this into account. Falcon Engineering has developed a core expertise in optimizing heat pump performance based on careful monitoring of the performance of several dozen large institutional geoexchange heat pump systems we’ve designed. We’ve aggregated a lot of lessons learned expertise.
Case Study
In particular cases, such as the Canyon Falls Middle School in Kelowna, our team implemented geoexchange technology to heat and cool the school. The system consists of a network of 24 boreholes drilled deep into the underlying bedrock to a depth of 600 feet. Along with geoexchange, the school was equipped with solar PV panels, LED lighting, occupancy based controls, and a network automation system to reduce the energy use intensity of the school.
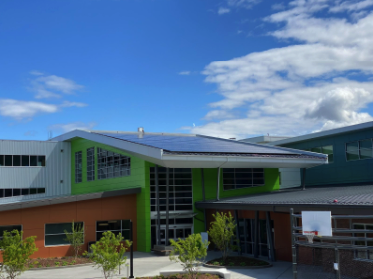
The net result is a near elimination of carbon emissions relating to the operation of this school. In this case, Falcon Engineering Ltd. provided mechanical, electrical, and energy system engineering services including energy modelling and geoexchange system design.
Conclusion
As a leader in green, energy efficient building systems, Falcon Engineering is well-suited to identify and implement low carbon energy solutions, including geoexchange where it is suitable, or identify other suitable low carbon alternatives for specific buildings in specific settings. At Falcon Engineering, our clients demand low carbon energy strategies to help guide their decision-making today, for achieving compatibility with tomorrow’s expectations. Reach out to us to speak with our highly qualified team of engineers, scientists and technical analysts about your geoexchange needs.
Find more information about our services here.
Want to learn more about energy engineering? Check out some of our other blogs!